There is a tripartite synergy between the science reach of the Forward Physics Facility (FPF) and astroparticle physics. Firstly, measurements at the FPF will probe high-energy hadronic interactions in the far-forward region. These measurements will improve the modeling of high-energy hadronic interactions in the atmosphere, reduce the associated uncertainties of air shower measurements, and thereby help to understand the properties of the highest energetic cosmic rays. Secondly, measurements at the FPF will help to understand the atmospheric neutrino flux and reduce the associated uncertainties on the main background for searches of high-energy astrophysical neutrinos with large-scale neutrino telescopes. Last but not least, FPF searches for unstable light new species characterizing rich hidden sectors will be complementary to dark matter indirect detection strategies.
High-energy collisions at the High-Luminosity Large Hadron Collider will produce an enormous flux of hadrons along the beam collision axis. On decay, these hadrons would yield intense, strongly collimated, and highly energetic beams of both neutrinos and antineutrinos of all three flavors. Dedicated measurements of the neutrino flux at the FPF will help to better understand light hadron production in the far-forward region. In particular, the ratio of electron to muon neutrino fluxes is a proxy for the ratio of charged kaons to pions. The kaon-to-pion ratio measured at the FPF experiments would in turn provide a direct test of possible strangeness enhancement in the far-forward region. The interest in this measurement is twofold. On the one hand, the ALICE collaboration has reported evidence for strangeness enhancement at mid-rapidity. On the other hand, a strangeness enhancement could help accommodate measurements of cosmic ray air showers for which the number of registered muons at the surface of the Earth is by some tens of percentage points higher than expected with extrapolations of existing hadronic interaction models.
High-energy (above about 1 TeV) atmospheric neutrinos produced in cosmic ray air showers are an irreducible background in searches for astrophysical neutrinos. Such a high-energy atmospheric neutrino flux is mainly produced via charm hadron decays. This provides a synergy between soft-QCD measurements and astroparticle physics. The production of charm quarks is dominated by gluon fusion. Particularly, gluon recombination (gg -> g) is expected to be relevant for x ~ 10^(-7) and so could tame the growth of the parton distribution function in this region. As shown in the figure, the FPF unprecedented statistics to probe gluon saturation in the far-forward region will provide important information to reduce the uncertainties and strongly constrain models of charm hadron production. This in turn will have a significant impact on the searches for astrophysical neutrinos by reducing the associated uncertainties of the high-energy atmospheric neutrino flux.
Indirect dark matter detection is driven by searches of an anomalous flux of photons, neutrinos, or cosmic rays produced in annihilations or decays of dark matter candidates gravitationally accumulated in celestial objects. A key point of indirect dark matter searches is to look for channels and ranges of energy where it is possible to beat the background from ordinary astrophysical processes. FPF experiments will provide complementary search strategies for unstable light new species characterizing rich hidden sectors in which dark matter production in the early Universe occurs differently than it would be dictated by the vanilla freeze-out mechanism.
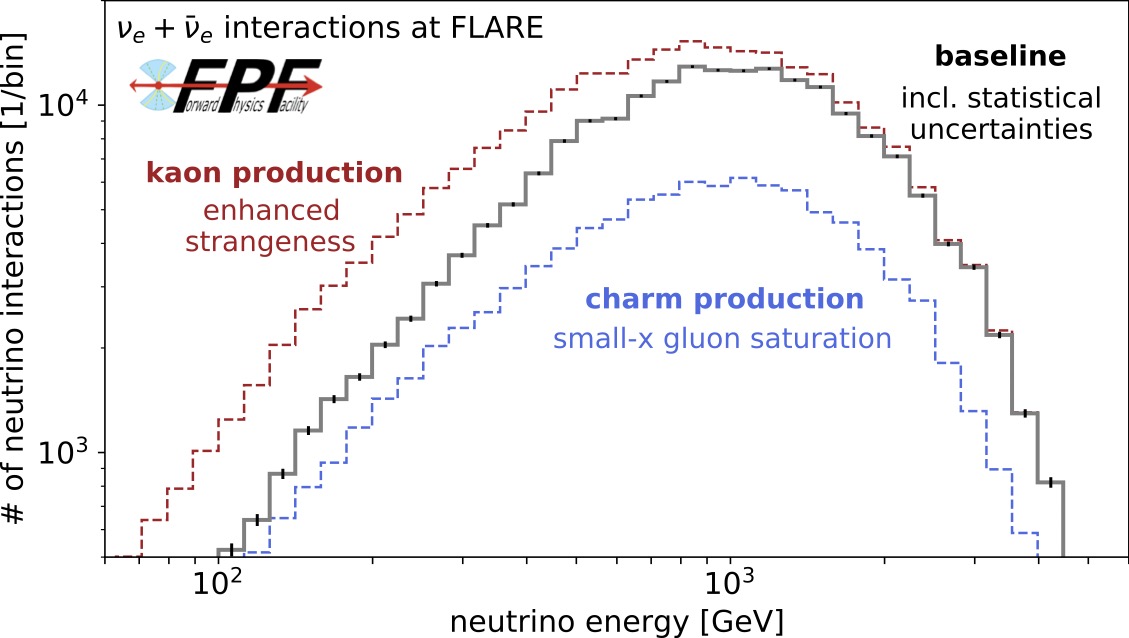